Quantum computing represents a revolutionary leap forward in computer science. It utilizes the unique principles of quantum theory to solve problems intractable for classical computers.
Unlike classical computers, which use bits as the smallest unit of information, quantum computers use quantum bits, or qubits, which can exist in multiple states simultaneously.
This allows quantum computers to process vast amounts of data at unprecedented speeds, opening up new possibilities for advancements in various fields such as cryptography, finance, healthcare, and artificial intelligence.
What is Quantum Computing?
Quantum computing is a cutting-edge area of computer science that leverages the principles of quantum theory to develop new computing methods.
Quantum theory explains the behaviour of energy and matter on the atomic and subatomic levels, where particles can exist in multiple states simultaneously.
This unique behaviour is harnessed in quantum computing to perform calculations at speeds and complexities far beyond the capabilities of classical computers.
In classical computing, information is encoded in either 0 or 1 bits. This binary approach limits the processing power of classical computers, as they can only perform calculations sequentially.
Quantum computing uses quantum bits or qubits. Qubits have the extraordinary ability to exist in multiple states simultaneously, thanks to the principle of superposition.
This means a qubit can represent both 0 and 1 simultaneously, exponentially increasing the computing power as more qubits are added.
Qubits can be entangled, a phenomenon where the state of one qubit is directly related to the state of another, no matter the distance between them.
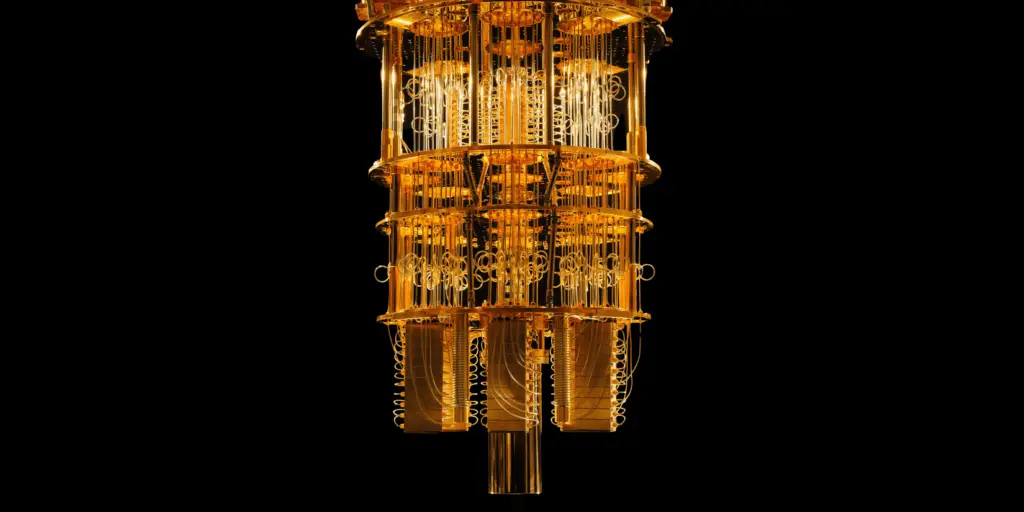
This entanglement enables quantum computers to solve complex problems more efficiently than classical computers.
The theoretical foundations of quantum computing were laid in the 1980s when it was discovered that some computational issues could be tackled more efficiently using quantum algorithms than classical ones.
Quantum computing exploits the interference patterns of wave-like quantum states, allowing it to process many possibilities simultaneously and extract solutions to highly complex problems.
Key Concepts
Superposition This allows qubits to be in a combination of all possible states at the same time. A quantum computer with multiple qubits in superposition can perform many calculations at once.
Entanglement: When qubits become entangled, the state of one qubit is directly tied to the state of another. This interconnectedness can be used to perform complex computations more efficiently.
Decoherence is a phenomenon in which the quantum state of qubits decays due to external interference, leading to errors in computation. Maintaining a stable quantum state requires isolating qubits from environmental factors through supercooled refrigerators and vacuum chambers.
Potential and Current Applications
Quantum computing has the potential to revolutionize numerous fields by solving problems that are currently unsolvable with classical computers.
- Enhancing data encryption and secure information sharing.
- Optimizing investment portfolios and improving fraud detection systems.
- Accelerating drug discovery and developing personalized medicine.
- Transforming machine learning by processing vast amounts of data more efficiently.
- Using advanced sensors for water cleanliness and pollution tracking.
As research and development continue, quantum computing is expected to unlock new capabilities and efficiencies across various industries, making it one of the most transformative technologies of the 21st century.
Key Features of Quantum Computing
Quantum computing is based on several unique features derived from the principles of quantum mechanics, which allow it to perform computations far more efficiently than classical computers.
One of the fundamental features is superposition. In classical computing, bits can be either 0 or 1. Qubits in a quantum computer can exist in a state of superposition, where they represent both 0 and 1 simultaneously.
This property allows quantum computers to process many possibilities simultaneously, significantly enhancing their computational power.
Entanglement is another critical feature of quantum computing. When qubits become entangled, the state of one qubit is directly linked to the state of another, regardless of the distance between them.
This interconnection enables quantum computers to perform complex calculations more efficiently than classical computers. Quantum algorithms leverage this entanglement to solve problems infeasible for classical systems.
Decoherence is a challenge unique to quantum computing. It occurs when the quantum state of qubits is disturbed by external factors such as vibrations or temperature changes, leading to errors in computation.
To mitigate decoherence, quantum computers are often kept in highly controlled environments, such as supercooled refrigerators, to maintain the stability of qubits during calculations.
Quantum computers also differ significantly from classical computers in how they process information. Classical computers increase their processing power linearly by adding more bits.
Quantum computers experience an exponential increase in power by adding more qubits.
This exponential growth means that even a few qubits can enable a quantum computer to perform calculations that would take classical computers millions of years to complete.
The interplay between superposition, entanglement, and decoherence defines quantum computing’s extraordinary potential and current limitations.
As these technologies advance and researchers find ways to manage decoherence and other challenges, the power and efficiency of quantum computers are expected to revolutionize fields such as cryptography, artificial intelligence, and complex system simulations.
How Quantum Computing Works
Quantum computing operates on principles derived from quantum mechanics, fundamentally differing from classical computing in processing information.
Qubits are the quantum analogue of classical bits at the heart of quantum computing. Unlike classical bits, which can be either 0 or 1, qubits can exist in a superposition state, meaning they can be both 0 and 1 simultaneously.
This ability allows quantum computers to process many possibilities concurrently, providing them immense computational power.
Superposition is achieved by placing qubits in a quantum state where they simultaneously represent multiple combinations of 0 and 1.
This is typically done using particles such as electrons or photons, which can be manipulated into superposition using energy pulses like lasers.
Another essential feature of quantum computing is entanglement. When qubits become entangled, the state of one qubit is intrinsically linked to the state of another, no matter the distance separating them.
This entanglement enables quantum computers to perform complex calculations more efficiently by exploiting the correlations between entangled qubits.
Changes to one qubit will instantly affect the entangled partner, allowing for coordinated and highly efficient computations.
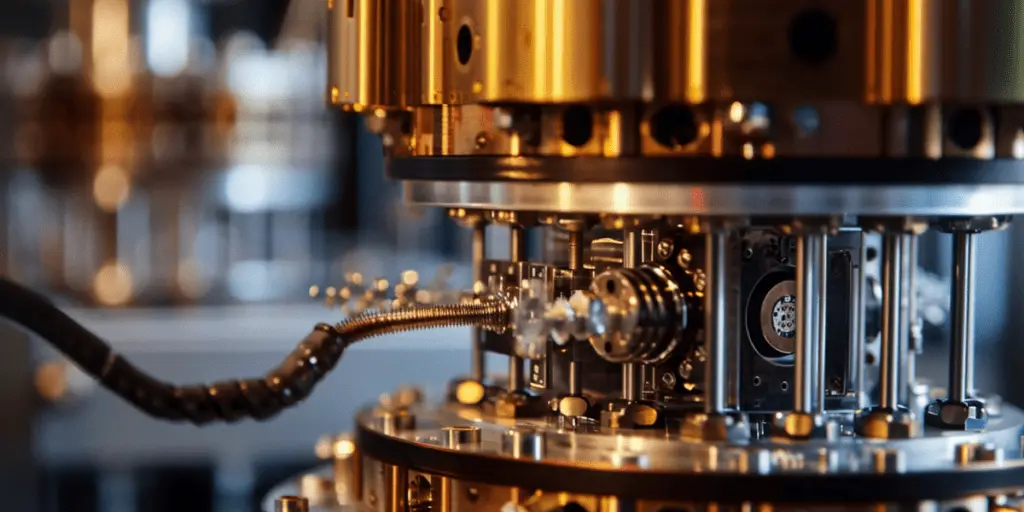
Quantum algorithms are designed to leverage the properties of superposition and entanglement.
These algorithms work by setting up a quantum system in superposition and then manipulating the system so that the correct answer emerges through constructive interference of quantum states.
Decoherence is a significant challenge in quantum computing. It refers to the loss of quantum coherence, where qubits lose their quantum behaviour and revert to classical states due to environmental disturbances such as thermal vibrations or electromagnetic fields.
This process can introduce errors into computations, making it crucial to isolate qubits from all forms of external interference.
This is often achieved using extreme cooling techniques, such as dilution refrigerators, which can bring temperatures to absolute zero, and employing vacuum chambers to reduce interference.
The structure of a quantum computer typically includes a set of qubits, control mechanisms to manipulate the quantum states, and a classical computer to run programs and interpret the results.
The qubits are maintained in controlled environments to ensure stability and coherence throughout the computation process.
Benefits of Quantum Computing
Quantum computing offers transformative potential across various fields due to its unparalleled processing capabilities. One of the most significant benefits is in the realm of cryptography and security.
Quantum computers can enhance data encryption techniques, making information sharing more secure. They can also break existing encryption methods, necessitating the development of new cryptographic protocols.
In finance, quantum computing can revolutionize portfolio management by optimizing investment strategies more effectively and efficiently than classical computers.
Financial institutions can leverage quantum algorithms to create better trading simulators and improve fraud detection systems, ultimately leading to more secure and profitable operations.
The healthcare industry stands to gain immensely from quantum computing. Simulating molecular structures at a quantum level can accelerate drug discovery and the development of new treatments.
Quantum computing can also facilitate personalized medicine by enabling more advanced genetic analysis and targeted medical interventions.
Quantum computing’s ability to solve complex optimization problems can significantly benefit the aerospace and manufacturing sectors.
It can be used to design more efficient and safer aircraft, optimize traffic planning systems, and improve manufacturing processes through advanced simulations and modelling.
Quantum computing can also enhance environmental monitoring and sustainability efforts. Quantum sensors can detect and monitor pollutants more accurately, helping maintain water cleanliness and environmental health.
Quantum computing can contribute to advancements in renewable energy technologies, such as more efficient solar cells and energy storage solutions.
Artificial intelligence and machine learning are other areas where quantum computing can profoundly impact.
Quantum algorithms can process and analyze vast amounts of data more quickly, leading to more sophisticated AI models and faster training times.
This can drive innovations in various applications, from natural language processing to autonomous vehicles.
Quantum Computers vs. Classical Computers
Feature | Quantum Computers | Classical Computers |
Basic Unit of Information | Qubit | Bit |
State | Can be in superposition | Can be either 0 or 1 |
Processing Power | Grows exponentially with the addition of qubits | Grows linearly with the addition of bits |
Core Principles | Quantum mechanics | Boolean logic and classical physics |
Error Rate | Higher error rates due to decoherence and quantum noise | Low error rates with established error correction techniques |
Environmental Requirements | Requires extremely low temperatures and isolation from interference | Operates at room temperature with basic cooling systems |
Ideal Use Cases | Complex simulations, cryptography, optimization problems | Everyday computing tasks, simple calculations |
Data Storage | Limited by current qubit technology, data retrieval can cause errors | Reliable and well-established digital storage methods |
Algorithm Efficiency | Superior for specific quantum algorithms | Efficient for a wide range of classical algorithms |
Development Stage | Experimental and early-stage commercial systems | Mature and widely used across all sectors |
Cost | Very high, millions to billions of dollars | Relatively low and affordable for consumers and businesses |
Speed | Potential to solve certain problems exponentially faster | Limited by Moore’s law, slower for complex problems |
Maintenance | Requires advanced maintenance for stability and coherence | Standard maintenance, widely understood |
Error Correction | Complex, still under development | Established, efficient error correction methods |
Practical Applications | Currently limited, potential in AI, healthcare, cryptography | Wide-ranging from personal use to enterprise applications |
Challenges and Limitations
While promising immense potential, quantum computing faces several significant challenges and limitations that must be addressed to realize its full capabilities.
One of the primary challenges is decoherence. Decoherence occurs when the quantum state of qubits is disrupted by external factors such as thermal vibrations, electromagnetic fields, or other environmental interferences.
This disruption can cause qubits to lose their quantum properties, leading to errors in computations.
Maintaining the quantum state of qubits requires highly controlled environments, often involving supercooled refrigerators to keep temperatures close to absolute zero and vacuum chambers to isolate them from external influences.
Error correction is another major hurdle in quantum computing. Due to their quantum nature, qubits are susceptible to more complex errors, unlike classical bits, which can benefit from straightforward error correction techniques.
A single mistake in a quantum computation can cascade and render the entire computation invalid.
While there has been progress in developing quantum error correction algorithms, such as using multiple qubits to encode and protect information, these solutions are still in their infancy and require further refinement to be practical on a large scale.
The current hardware limitations are also a significant barrier. The number of qubits available in quantum computers is relatively low, with leading systems only boasting a few hundred qubits.
Systems with thousands or even millions of qubits are necessary to solve more complex problems and realize the full potential of quantum computing.
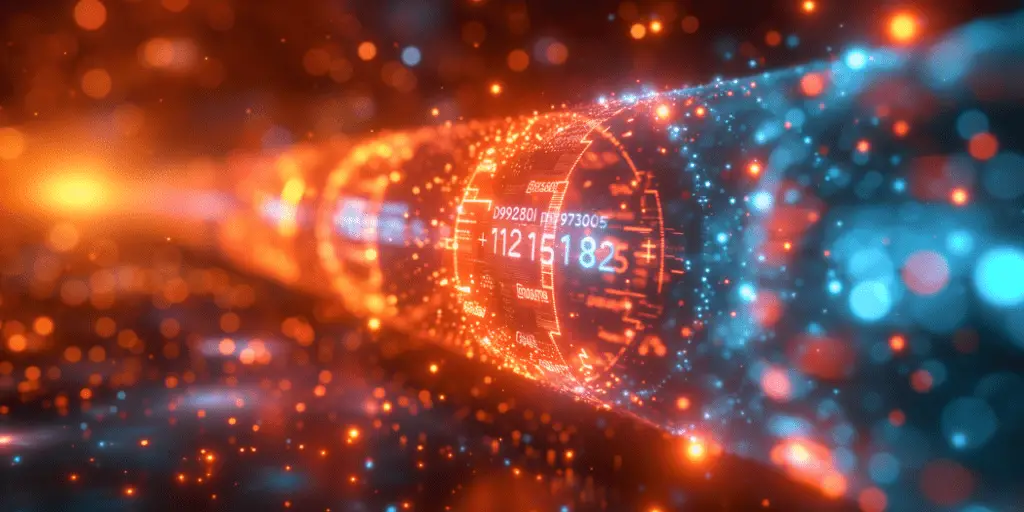
Developing stable and scalable quantum processors with many qubits remains a formidable engineering challenge.
Quantum computers also face significant technical and environmental requirements. They must be kept at extremely low temperatures to maintain qubit stability and coherence, necessitating sophisticated and expensive cooling systems.
These systems must be shielded from the slightest vibrations and electromagnetic interferences, further complicating their design and maintenance.
Due to these technical challenges, quantum computing’s practical applications still need to be improved. While quantum algorithms have shown theoretical promise in cryptography, optimization, and simulation, practical implementations are still experimental.
Quantum computers are currently best suited for specific problems where they can outperform classical computers.
Future Prospects
The future of quantum computing holds immense promise. It has the potential to revolutionize numerous fields by solving problems currently beyond the reach of classical computers. As technology advances, several key developments and prospects can be anticipated.
One of the most significant future prospects is the continued increase in qubit count and stability. Researchers are striving to develop quantum processors with thousands or even millions of qubits, which will dramatically enhance quantum computers’ computational power and capabilities.
Achieving this milestone will enable quantum systems to tackle more complex and larger-scale problems, pushing the boundaries of what is computationally possible.
Advancements in error correction techniques are also crucial for the future of quantum computing. Improved algorithms and methods to mitigate decoherence and quantum noise will enhance the reliability and accuracy of quantum computations.
As error rates decrease, the practical applications of quantum computing will expand, making it a more viable and dependable technology for a wide range of industries.
Another exciting prospect is the integration of quantum computing with other emerging technologies, such as artificial intelligence and machine learning.
Quantum algorithms can accelerate AI training and optimization, leading to more sophisticated and efficient AI systems. This synergy could drive innovation in various sectors, including healthcare, finance, and autonomous systems.
Quantum computing as a service is expected to become more prevalent, allowing organizations to access quantum computing resources via the cloud.
This model will democratize access to quantum computing, enabling smaller businesses and research institutions to leverage its power without significant upfront investments in hardware. Amazon, Microsoft, and IBM are already paving the way with their cloud-based quantum services.
Government and private sector investments in quantum research and development will likely continue to grow. National initiatives and collaborations between academia, industry, and government agencies will be pivotal in advancing quantum technologies.
These efforts will drive breakthroughs in quantum algorithms, hardware, and applications, further accelerating the development and adoption of quantum computing.